Can Monkeys Learn To Drive? Absolutely, and LEARNS.EDU.VN explores this fascinating question, revealing surprising insights into primate cognition and spatial learning. This exploration highlights the remarkable adaptability of monkeys and offers a glimpse into the shared learning systems across species, providing a fascinating look at animal behavior and cognitive flexibility. We’ll delve into the fascinating intersection of primate cognition, animal behavior, and spatial awareness.
1. Introduction: Unveiling Primate Spatial Navigation
Spatial learning, the ability to acquire, integrate, and retrieve spatial information, is crucial for navigating our environment. This involves finding objects, recalling locations, and moving through the world effectively. To achieve this, our brains create and update “cognitive maps” in real time, using either external coordinate systems or internal frames of reference. This complex process, foundational to spatial awareness and understanding, highlights the importance of spatial cognition in everyday life.
Humans aren’t the only ones who rely on spatial learning. Animals, too, navigate using various strategies that depend on factors like training duration. Research has illuminated the connection between allocentric (place-based) and egocentric (response-based) learning strategies. A foundational study by Packard and McGaugh demonstrated how rats adapt their navigation based on experience, shifting between different strategies over time. This highlights the flexibility and adaptability of learning systems in animals and humans alike.
1.1. Bridging the Gap: Spatial Navigation in Primates vs. Rodents
While the rodent brain contains specialized “place cells” that fire in specific locations, their primate counterparts appear to be more closely linked to visual orientation. This difference raises important questions about the universality of spatial navigation mechanisms across species. Moreover, the limited number of studies conducted on primates in real-world environments further complicates our understanding. A deeper exploration of these topics is possible with LEARNS.EDU.VN.
1.2. Real-World Research: A Novel Approach to Primate Navigation
Current research limitations include a reliance on virtual environments (VE) or restrained settings for studying primate navigation, which can hinder the integration of crucial idiothetic cues (internal cues). Additionally, many studies focus on comparing only one non-spatial strategy (direction or cue-based) with the place strategy, often overlooking the simultaneous comparison of all three alternatives. Understanding these dynamics is crucial for a comprehensive understanding of spatial learning.
To address these gaps, LEARNS.EDU.VN will explore a pioneering task designed to study primate spatial navigation in real-world environments. This innovative approach will allow researchers to conduct pharmacological and electrophysiological procedures, providing valuable insights into the neural mechanisms underlying spatial learning. This exploration will uncover the remarkable adaptability of primates and offer a fascinating glimpse into the shared learning systems across species, making it a crucial step in comparative cognitive research.
2. Materials and Methods: Setting the Stage for Discovery
2.1. Experimental Subjects: Rhesus Monkeys as Navigators
Three female rhesus monkeys (Macaca mulatta) participated in the study. They were housed under standard conditions with a 12-hour light/dark cycle, controlled humidity (60%), and temperature (22°C ± 2°C). A veterinarian specializing in non-human primate care oversaw all aspects of animal welfare. Experimental procedures adhered to the Council Directive of 2010 (2010/63/UE) of the European Community and the National Institute of Health Guide for the Care and Use of Laboratory Animals.
The Ethical Committee for Animal Research CE50 approved the protocol (Agreement number: 5012078-A). The monkeys were fed once daily and enjoyed an enriched environment with mirrors, Kong toys, and Primatec FP3 candies. Water consumption was limited during experimental days but provided ad libitum on non-working days. Body weights were monitored weekly and maintained at a stable level throughout the experiments. This rigorous ethical and care framework ensures the well-being of the animals while allowing for groundbreaking research.
2.2. Behavioral Task: Designing the Monkey Maze
2.2.1. Maze Design: Creating a Navigable Environment
A 3×3 room maze was constructed in a room that could be blacked out with heavy curtains (Figure 1). The maze featured a smooth chipboard floor and 180 cm high walls made of 24 mm chipboard. Each room measured 150×150 cm, and walls and floors were painted matte black. A 70 cm gap surrounded the maze’s exterior. Door openings were 70 cm wide, and a set of two 7-bar LED panels (5 cm high) was placed on the left of each door opening.
Physical doors were avoided to facilitate wheelchair movement. During experiments, the room was completely dark, illuminated only by the LED panels within the monkey’s current maze section. This design ensured the monkeys could not see from one section to another, enhancing the focus on the illuminated cues. A central computer controlled the LED panels from a screened area, providing precise management of the visual stimuli.
2.2.2. Pre-training: Mastering the Wheelchair
Monkeys first learned to drive a motorized wheelchair (Action Junior 3, Invacare; Figure 2A). Initially, they were trained to use a joystick with their right hand to obtain water rewards. They then became accustomed to sitting in the wheelchair, controlled by a joystick on the right armrest, with a restraining seat ensuring they remained in the chair during testing. Progressively, the monkeys were required to move the chair forward to reach a water syringe held by an experimenter at various positions within a room.
Next, the monkeys were placed in the maze and guided through a series of rooms by the trainer to receive rewards. They were then trained to navigate the dark maze, with all symbols in the current room illuminated. Finally, they learned to follow a route from room to room by selecting the door with the illuminated panel (Figure 2B). Monkeys advanced from the pre-training phase once they demonstrated comfort with driving the wheelchair, avoiding wall collisions, making sequences of turns in all directions, and maintaining forward trajectories. They also had to master dark navigation, using illuminated cues to complete pathways in each room.
2.2.3. Navigation Task: Learning the Route
Each session comprised an acquisition phase, where the monkey learned a route through the maze, followed by a probe phase, where the starting position or final decision point cues were altered.
During the acquisition phase, monkeys had to learn a sequence of three choices to navigate a route through four rooms. Each decision point required the monkey to drive the wheelchair through a door opening into the next room. At the start of each trial, the monkey was placed in the start room, and the LED panels illuminated for 2 seconds. Subsequently, only the symbol above the door leading to the next room in the required sequence remained lit.
If the monkey chose the correct illuminated door, the panel in the previous room was extinguished, and the panels in the new room lit for 2 seconds, with one cue then indicating the next direction. Correctly following the four-room sequence resulted in a water reward in the final room. If the monkey made an error by passing through a non-illuminated door, all panels were extinguished, and the monkey was returned to the start room by the handler for the next trial. After both successful and unsuccessful trials, the handler returned the animal to the same start room in the dark via a randomly chosen route to minimize the development of a cognitive map during the inter-trial period.
The start room and correct sequence of rooms remained constant throughout an acquisition block, but changed for each new block. Two types of acquisition blocks were used, differing only in the number of consecutive successful trials required to meet the criterion: short training blocks required 3 successful trials, while long training blocks required 10. Each monkey performed at least one short block and one long block each day, in random order. A maximum of 25 trials was allowed. If a monkey did not meet the criterion by the 25th trial, the pathway was changed, or the animal was returned to its housing place, depending on experimental conditions and the monkey’s compliance. Upon meeting the criterion, a probe test was conducted to determine the spatial strategy used, with one of four different probe tests administered after each acquisition block. Over twenty acquisition sessions and linked probe tests were conducted for each monkey and each acquisition criterion. Acquisition performance (trials and errors to meet the criteria) was compared between the first 10 and the last 10 sessions.
2.2.4. Probe Tests: Deciphering Spatial Strategies
The probe tests were designed to distinguish between different navigation strategies, allowing researchers to pinpoint how monkeys were making decisions.
- Type 1: Triple Dissociation (Figure 1A&B): During the acquisition phase (Figure 1A), the start room and reward room remained consistent, and the directions were signaled with the same cue on every trial. The final choice always involved the same direction and cue. In the probe trial (Figure 1B), both the start room and the final cue position were changed. The monkey followed the same cues in the same directions as in the acquisition phase for the first two decision points. At the final decision point (center room), three possibilities arose: returning to the room where the reward was received during the acquisition phase (place strategy), turning left as in the acquisition phase (direction strategy), or following the cue (cue strategy).
With only two different durations of acquisition, it was not possible to evaluate the order of all three strategies with respect to speed of learning. Therefore, the following three probes were designed to test the strategies pairwise.
- Type 2: Place versus Cue (Figure 1C&D): After the acquisition phase (Figure 1C), the start position was changed. At the final decision point (Figure 1D), the animal had to choose between returning to the same room where the reward was given during the acquisition phase (place strategy) or following the cue that had led to the reward during acquisition (cue strategy).
- Type 3: Place versus Direction (Figure 1E&F): The animal had to choose between returning to the same room where the reward was given during the acquisition phase (place strategy) or following the direction that had led to reward during acquisition (direction strategy).
- Type 4: Cue versus Direction (Figure 1G&H): The animal had to choose between repeating the same direction of turn as in the acquisition (direction strategy) or following the cue that had led to the reward during acquisition (cue strategy).
2.3. Data Analysis: Quantifying Monkey Choices
In the acquisition phase of each block of trials, the number of errors and trials to reach the criterion (3 or 10 correctly executed trials in succession) were recorded. For the probe phase, the distribution of choices (cue, place, or direction) was recorded. Paired Wilcoxon Sign Rank, Fisher’s exact, and Chi-square’s tests were used to analyze monkey’s performances, distribution of choices in probe trials, and the effect of the acquisition’s length, respectively.
Comparisons between monkeys’ choices are summarized in Table 1. Data from all monkeys were pooled when no significant difference was found between them. A p-value of 0.05 was used as the level of significance throughout this study.
Table 1: Monkeys’ choices comparison.
Probe Test | Short Blocks | Long Blocks |
---|---|---|
Place vs Direction | 0.30 | 0.194 |
Direction vs Cue | 0.129 | 0.654 |
Cue vs Place | 0.502 | 0.311 |
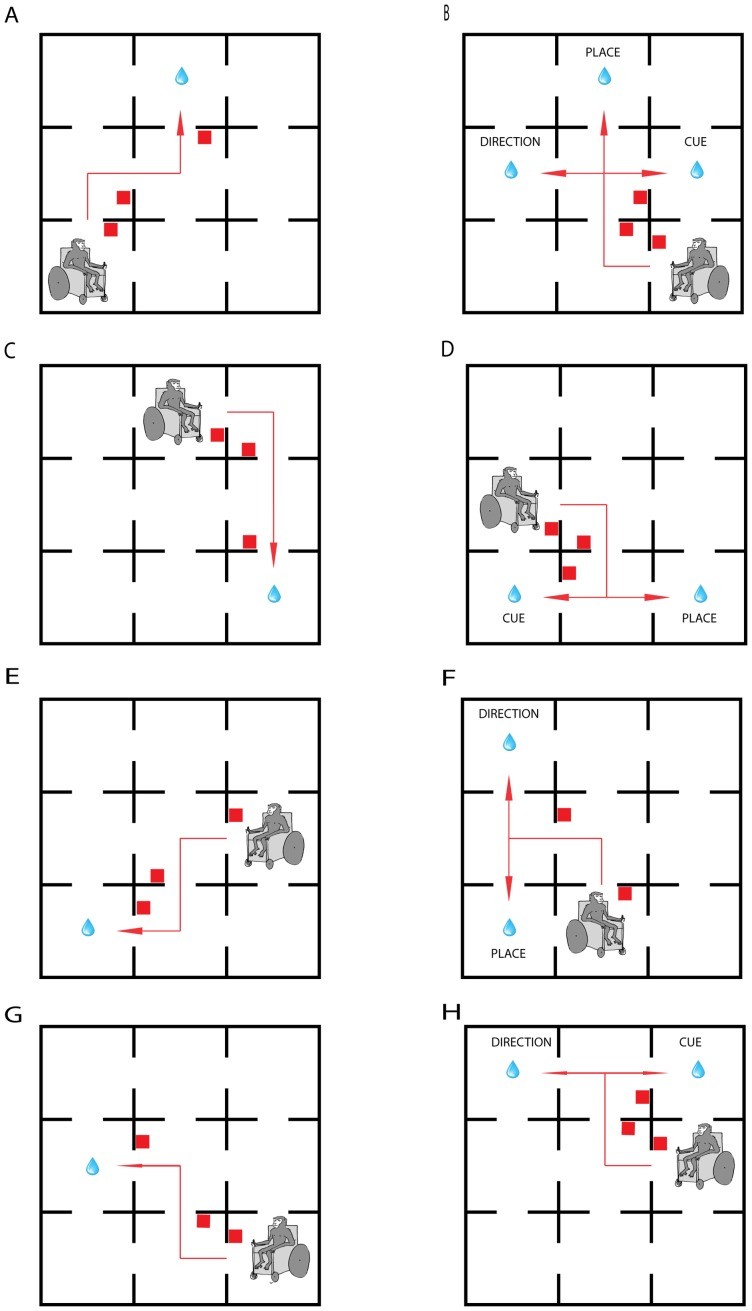
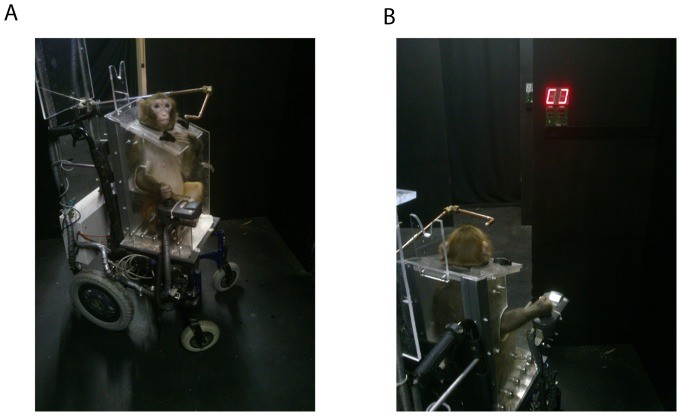
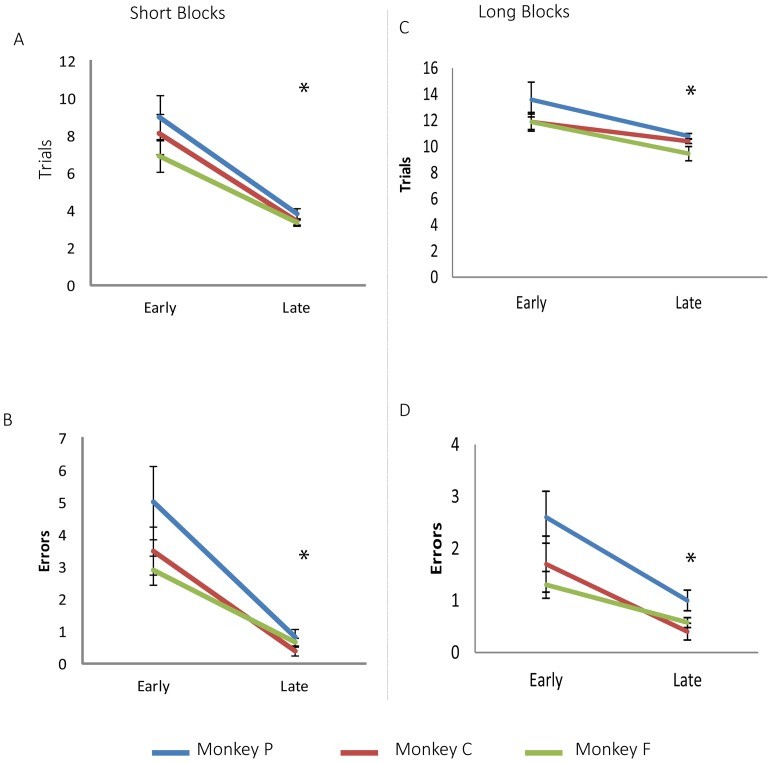
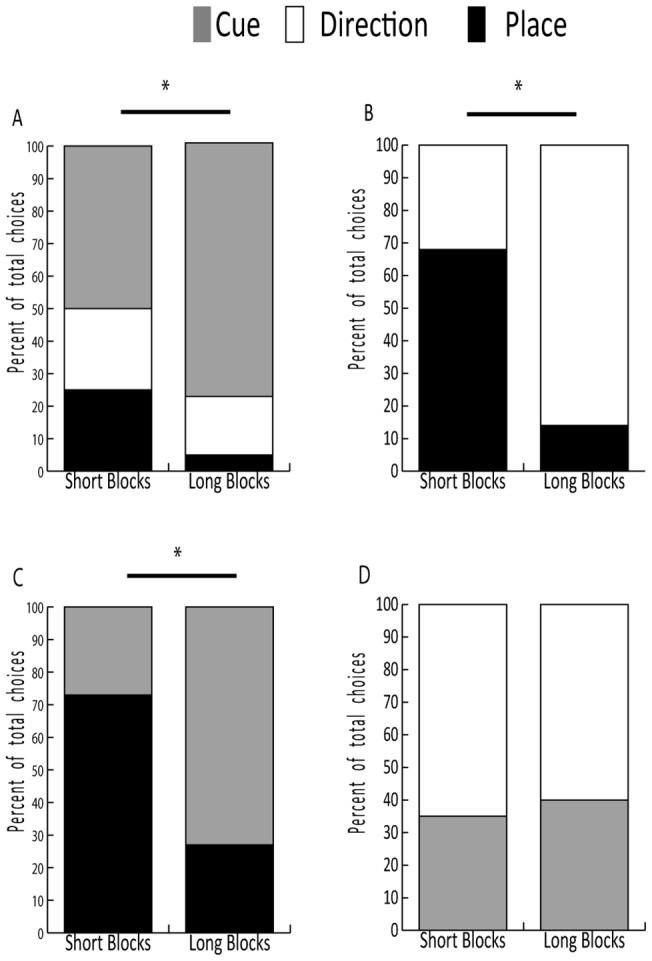
3. Results: Unveiling Primate Navigation Strategies
3.1. Performance Improvement: Learning Curves in Action
All monkeys successfully learned to operate the powered wheelchair and navigate a path through the maze to obtain rewards. The monkeys’ learning performance improved with training, as evidenced by a decrease in the number of trials and errors required to meet the criteria in both short and long acquisition blocks (Figure 3). No significant differences were found between individual monkey performances (Table 2).
Table 2: Monkeys’ learning comparison.
Acquisition length | Early Training | Late Training |
---|---|---|
Trials | Errors | |
Short Blocks | 0.376 | 0.238 |
Long Blocks | 0.91 | 0.212 |
3.2. Consistency Across Subjects: No Significant Individual Differences
There were no significant differences between the monkeys’ choice distributions in the different probe tests (Table 2), indicating a consistent approach to learning and navigation among the subjects. This consistency allows for the pooling of data to draw more robust conclusions about the overall strategies employed.
3.3. Dissociating Strategies: Unraveling the Navigation Toolkit
After reaching the criterion in an acquisition block, a probe test was conducted to identify the navigation strategy used by the animals.
3.3.1. Type 1: Triple Dissociation
In the Type 1 probe, animals had three choices at the final decision point (Figure 1B). After short acquisition blocks, no preference was shown among the three possible final choices. However, after long acquisition blocks, the animals significantly favored the cue strategy (78%) over direction or location strategies (χ2 = 8.235, p = 0.016, Figure 4A).
3.3.2. Type 2: Place versus Cue
In the Type 2 probe, the cue at the final decision point was moved, leaving the animal with only two possible choices. Navigation strategy differed significantly based on the acquisition duration. After short blocks, animals more often returned to the room rewarded during acquisition (place strategy), whereas after long blocks, they favored the cue strategy (χ2 = 22.891, p < 0.001, Figure 4B).
3.3.3. Type 3: Place versus Direction
In the Type 3 probe, no cue symbols were illuminated at the final choice point, so animals could not use a cue strategy (Figure 1F). After short acquisition blocks, animals used a place strategy more often than a direction strategy. After long acquisition blocks, animals used a direction strategy more frequently (χ2 = 36.821, p < 0.001, Figure 4C).
3.3.4. Type 4: Cue versus Direction
In the final probe type, animals could not return to the place where they were rewarded during acquisition because this would have required a diagonal movement. When only cue and direction choices were available (Figure 1H), the monkeys preferentially used a direction strategy. No significant difference was found between choices made after short and long acquisition blocks (χ2 = 0.117, p = 0.733, Figure 4D).
4. Discussion: Interpreting Primate Navigation Strategies
These findings confirm that non-human primates can learn to navigate a powered wheelchair through a real-world environment and utilize visual cues to guide their movements. The success in optimizing navigation during the acquisition phase, with minimal errors before meeting the criteria, highlights the adaptability of primates to this task. This exciting study has great implications, and LEARNS.EDU.VN is committed to communicating them in an accessible way.
4.1. Unveiling Three Distinct Strategies: Place, Direction, and Cue
Careful manipulation of the probe phase revealed three distinct spatial navigation strategies used by the monkeys: returning to the rewarded location (place strategy), repeating the sequence of body turns that led to the reward room (direction strategy), and following the cue that led to the rewarded location (cue strategy). In the first probe variant (Figure 1A&B), the cue was presented in a position different from its acquisition phase position, and the start position was changed. By forcing the final decision point to be made in the center room, the monkey had three possible choices, each implying a different strategy: place, direction, or cue.
The triple dissociation probe type’s results in the short acquisition blocks did not reveal any significant preference for any navigation strategy. This may indicate the complexity of the task, where the cognitive map formed after only three consecutive correct acquisition trials is not robust enough to handle both a changed starting position and a changed final decision point offering three alternative choices. It remains unclear whether the animal is attempting to use the cognitive map formed during the acquisition phase but encounters too much competition between strategies, or whether the animal re-enters an exploration phase to form a new cognitive map.
However, in the long blocks (after ten trials), the cue navigation strategy was preferred, suggesting an increased predictive value of the cue and heightened attention to external stimuli under uncertainty.
4.2. Pairwise Probe Tests: Confirming the Learning Order
Based on these observations, three other variants of the probe test were developed, each offering only two possible navigation strategies at the final decision point. These pairwise probe tests revealed the order in which the strategies were learned. The place vs. cue variant probe showed a dissociation of place and cue navigation (Figure 1C&D). After short blocks, animals preferentially returned to the room rewarded during acquisition using the place strategy, whereas after long blocks, all animals preferentially used the cue strategy. This suggests that the predictive value of the cue is learned more slowly than the value of a place, having a stronger influence on navigation strategy once learned.
The same predominance of place strategy use was observed after short blocks in the place vs. direction probe (Figure 1E&F) test. However, after long acquisition blocks, animals chose the direction that led them to the reward during the acquisition phase. In the final probe variety, cue vs. direction, all animals used a direction strategy after both short and long acquisition blocks.
4.3. Synthesizing the Strategies: A Hierarchical Model of Spatial Learning
Combining the results of these four probes suggests a hierarchical strategy where a place strategy dominates early in learning, followed by a cue/direction strategy later. In the triple dissociation probe, the cue strategy was dominant after long acquisition blocks, but when tested pairwise, no significant difference was found between the cue and direction strategies. Both direction and cue strategies can be considered “sequence-based” strategies, with the former represented by sequences of body turns and the latter relying on distinct (or sequences of) local landmarks.
These findings support the hypothesis that multiple, parallel neural systems are used for spatial learning. These systems learn at different rates, and the strategy used represents the system that arrives at a decision faster after a given length of training. This agrees with rodent studies demonstrating a transfer from goal-directed behaviors to habits involving activation of the dorsal striatum.
A recently published review of hippocampal/basal ganglia interactions in spatial navigation, based on experimental findings in rodents, suggests three parallel loops through the basal ganglia, one of which includes the dorsal hippocampus.
4.4. Implications for Primates: Mapping the Neural Circuits of Navigation
This study presents the first results showing a dissociation of three spatial navigation strategies in the non-human primate. Based on existing studies and the theoretical three-parallel loop architecture, the cue navigation strategy observed here could be supported by a loop through sensorimotor cortex and dorsolateral striatum, the direction strategy by a loop through prelimbic cortex and dorsomedial striatum, and the place strategy by a loop through entorhinal cortex and ventral striatum that includes the dorsal hippocampus.
4.5. Future Directions: Bridging the Gap Between Real and Virtual Worlds
The test bed presented here enables the investigation of several questions that have been impossible to address with current experimental paradigms. Firstly, most primate navigation tasks are performed in virtual reality environments. Comparing results from this task in real and virtual world environments may determine whether virtual world environments engage the same navigation strategies as the real world, and by extension, involve learning in the same brain areas. Despite a challenging study pointing out the presence of grid cells in the primate entorhinal cortex during a virtual navigation task, studying what occurs during real environment navigation is essential.
Two systems are known to have roles in building spatial knowledge: the hippocampal formation and the striatum. It is generally held that allocentric learning is related to the hippocampus, while the striatum is believed to be involved in egocentric representation. The role of the hippocampal formation in primates’ spatial navigation is less documented than in rodents, possibly due to the difficulty of designing protocols that allow for electrophysiological and pharmacological procedures with freely moving animals. Furthermore, basal ganglia (BG), and precisely the neostriatum, involvement in spatial navigation is increasingly studied. Given the striatum’s division into dorsolateral, dorsomedial, and ventral territories, conclusions about each territory’s involvement in spatial navigation remain unclear.
4.6. Applications in Neurodegenerative Disease Models
This test bed could investigate the spatial learning deficits associated with animal models of neurodegenerative diseases. Electrophysiological procedures with telemetry recording systems would be possible, allowing us to record neural activity throughout the 7×7 meter test room. Furthermore, inactivation of the basal ganglia in non-human primates leads to acute motor deficits. However, these animals can still manipulate a joystick, making it possible to study spatial navigation deficits in animal models of both Parkinson’s and Alzheimer’s diseases. Finally, this task should also be readily extensible to humans for translational studies.
Conclusion: The Road Ahead for Primate Navigation Research
This investigation into primate spatial navigation offers a compelling answer to the question: Can monkeys learn to drive? More importantly, it sheds light on the sophisticated cognitive abilities of primates, their capacity for learning, and the intricate neural mechanisms that underpin their spatial awareness. The study confirms that monkeys can indeed learn to drive and navigate through a maze, utilizing various strategies and adapting their approaches based on experience. This flexibility in spatial learning offers insights into the shared cognitive processes between primates and humans, enhancing our understanding of how the brain learns and navigates environments.
The research also underscores the importance of studying primate cognition in real-world environments to gain a more accurate understanding of their spatial learning abilities. This real-world approach, combined with advanced techniques, sets the stage for further exploration into the neural basis of navigation in primates and its relation to neurodegenerative diseases.
Discover more fascinating insights into animal behavior and primate cognition at LEARNS.EDU.VN. Explore detailed articles, participate in engaging courses, and expand your knowledge about the cognitive capabilities of animals and humans alike.
Call to Action: Expand Your Knowledge with LEARNS.EDU.VN
Are you fascinated by the cognitive abilities of animals and eager to learn more about spatial learning and navigation? Visit LEARNS.EDU.VN today to explore a wealth of resources, including detailed articles, engaging courses, and expert insights. Discover how you can enhance your understanding of animal behavior, primate cognition, and the shared learning systems across species. Join our community of learners and embark on a journey of intellectual discovery.
Contact Us:
- Address: 123 Education Way, Learnville, CA 90210, United States
- WhatsApp: +1 555-555-1212
- Website: LEARNS.EDU.VN
Frequently Asked Questions (FAQ)
1. Can monkeys really learn to drive?
Yes, monkeys can be trained to operate a motorized wheelchair and navigate through a structured environment like a maze. This study demonstrates their ability to learn and adapt using different spatial strategies.
2. What spatial navigation strategies do monkeys use?
Monkeys primarily use three strategies: place strategy (returning to a specific location), direction strategy (repeating a sequence of turns), and cue strategy (following visual cues).
3. How does training duration affect the navigation strategies used by monkeys?
With short training, monkeys tend to rely on place strategies. As training extends, they shift to using cue and direction strategies.
4. Why is it important to study primate navigation in real-world environments?
Real-world studies provide more accurate insights into how primates naturally navigate, as virtual environments may not fully replicate the cues and challenges of real-world navigation.
5. What role does the hippocampus play in primate spatial navigation?
The hippocampus is believed to be crucial for allocentric learning (place-based), while the striatum is involved in egocentric representation (direction-based).
6. Can this research help us understand human neurodegenerative diseases?
Yes, studying spatial learning deficits in animal models can provide valuable insights into diseases like Parkinson’s and Alzheimer’s, which affect spatial navigation abilities.
7. Are there differences in how rodents and primates use spatial cues?
Yes, rodents use place cells in the hippocampus to navigate, while primates’ hippocampal activity appears to be more closely linked to visual orientation.
8. How does LEARNS.EDU.VN contribute to this field of knowledge?
LEARNS.EDU.VN provides a platform for exploring these topics in depth, offering detailed articles, courses, and expert insights to enhance understanding of animal behavior and primate cognition.
9. What is the significance of the triple dissociation probe test in this study?
The triple dissociation probe test helps distinguish between the place, direction, and cue strategies used by monkeys when navigating. It reveals which strategy is favored under different conditions.
10. How can I learn more about the neural mechanisms behind spatial navigation in primates?
Visit learns.edu.vn to explore articles and courses that delve into the neural circuits and cognitive processes involved in spatial learning and navigation.