Which Statement Explains What Geologists Can Learn From The Graph? Understanding seismic data is crucial for predicting and mitigating earthquake risks, and LEARNS.EDU.VN provides comprehensive resources to help you master this skill. By analyzing graphical representations of seismic activity, geologists can glean invaluable insights into earthquake magnitude, energy release, and the geological composition of the Earth. Discover insightful educational content that empowers you with the knowledge to interpret seismic graphs effectively, understand earthquake science and master seismology.
1. Understanding Earthquake Magnitude: A Geologist’s Guide
Earthquake magnitude is a fundamental concept in seismology, representing the size or strength of an earthquake. It’s crucial to differentiate magnitude from intensity; magnitude is a single value per earthquake, while intensity varies geographically based on the shaking experienced. By examining graphs displaying seismic data, geologists can determine the magnitude of an earthquake and gain insights into the processes occurring deep within the Earth. This knowledge is vital for assessing seismic hazards and informing building codes.
1.1 Types of Magnitude Scales
Several magnitude scales exist, each with its own method of calculation and applicability:
- Richter Scale (ML): The most widely known scale, but primarily used for small, local earthquakes.
- Moment Magnitude (MW): The most accurate scale for all earthquake sizes, based on the physical properties of the earthquake.
- Energy Magnitude (Me): Measures the energy released by an earthquake, providing a different perspective on its size.
1.2 The Richter Scale (ML)
The Richter Scale, developed by Charles F. Richter in 1935, was a groundbreaking achievement in seismology. It provided a quantitative way to measure earthquake size based on the logarithm of the amplitude of waves recorded by seismographs. While originally designed for California earthquakes within 600 km of a specific seismograph, it laid the foundation for modern magnitude scales.
The Richter magnitude is determined by measuring the amplitude of seismic waves recorded on seismographs and then adjusting for the distance between the seismograph and the earthquake’s epicenter. This scale is particularly useful for local earthquakes where the seismograph is close to the epicenter.
1.3 Moment Magnitude (MW)
Moment Magnitude (MW) is now the standard scale for measuring earthquake size, especially for larger earthquakes. It’s based on the seismic moment (MO), which considers the rigidity of the rock, the area of the fault that slipped, and the distance the fault moved.
Moment (MO) = rigidity x area x slip
This calculation provides a more accurate representation of the earthquake’s physical effects. The formula to calculate MW is:
Moment Magnitude (MW) = (2/3) log10(MO) – 9.1
1.4 Magnitude Types Table
Magnitude Type | Magnitude Range | Distance Range | Equation | Description |
---|---|---|---|---|
Richter Scale (ML) | Typically < 7 | Local | Logarithmic amplitude measurement | Primarily used for small, local earthquakes. |
Moment Magnitude (MW) | No upper limit | Global | Based on seismic moment (rigidity, area, slip) | Most accurate for all earthquake sizes. |
Energy Magnitude (Me) | Varies | Global | Based on energy release | Measures the energy radiated by an earthquake. |
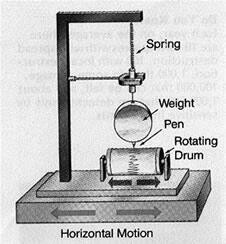
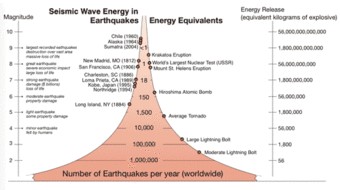
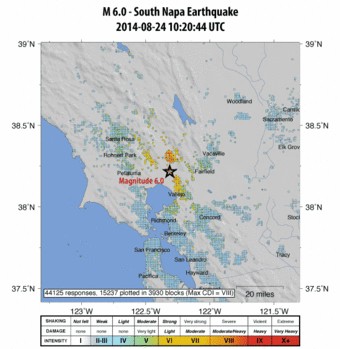
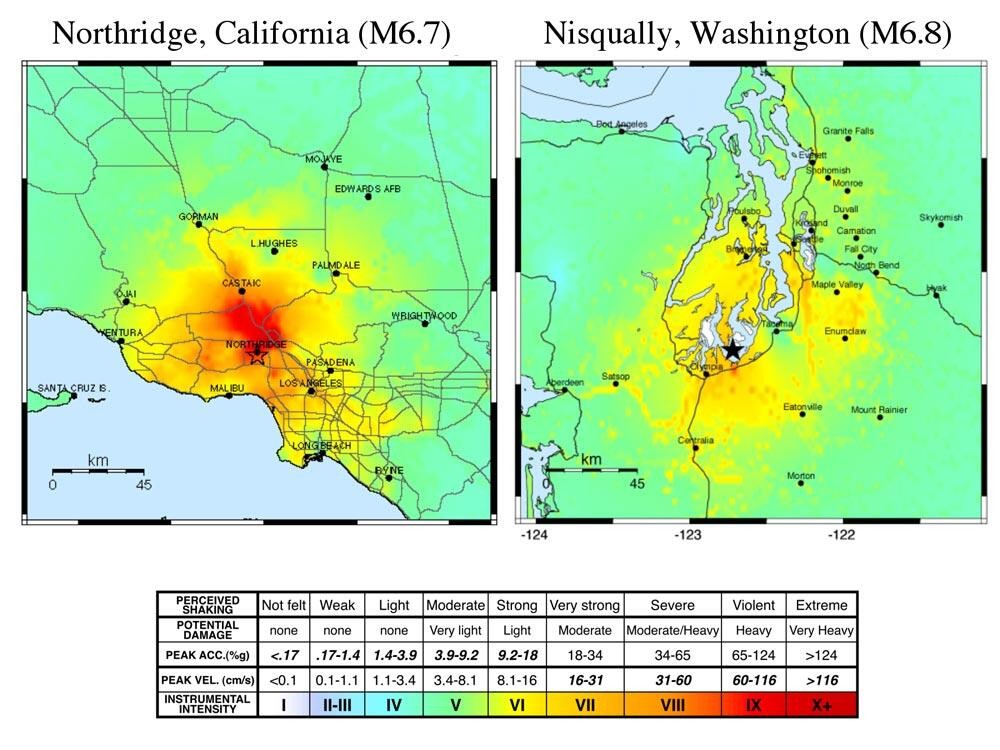
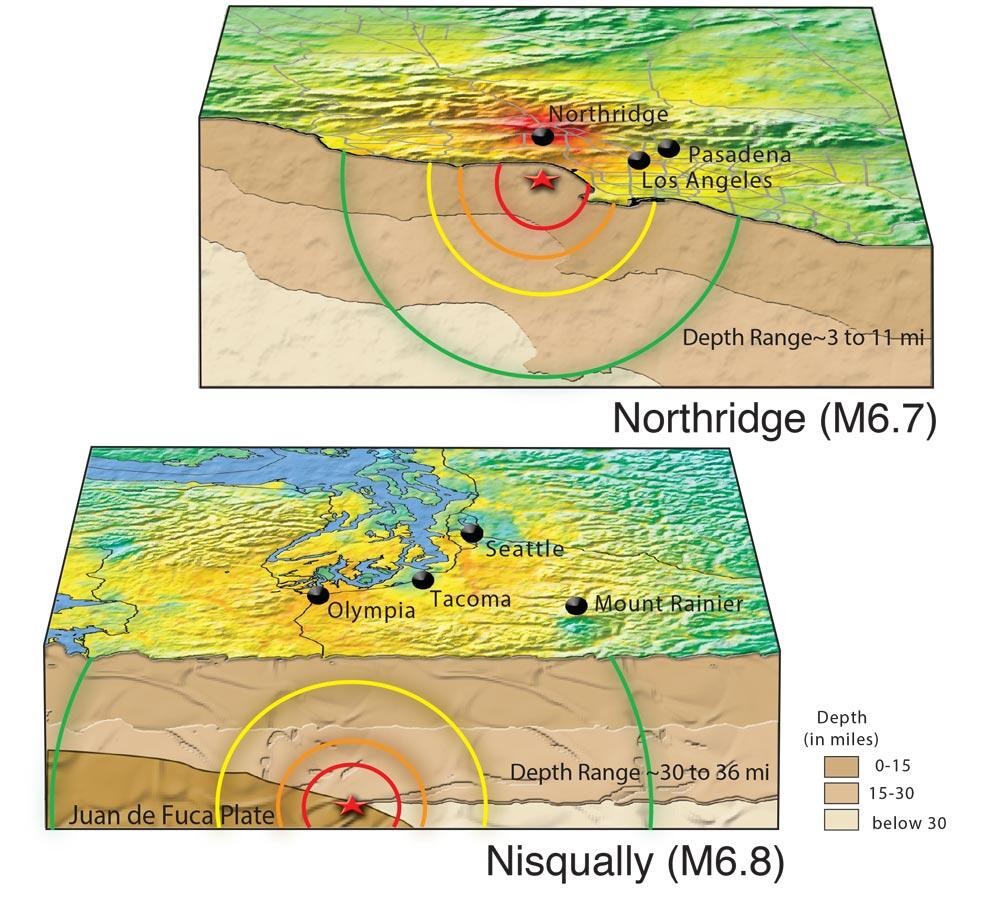
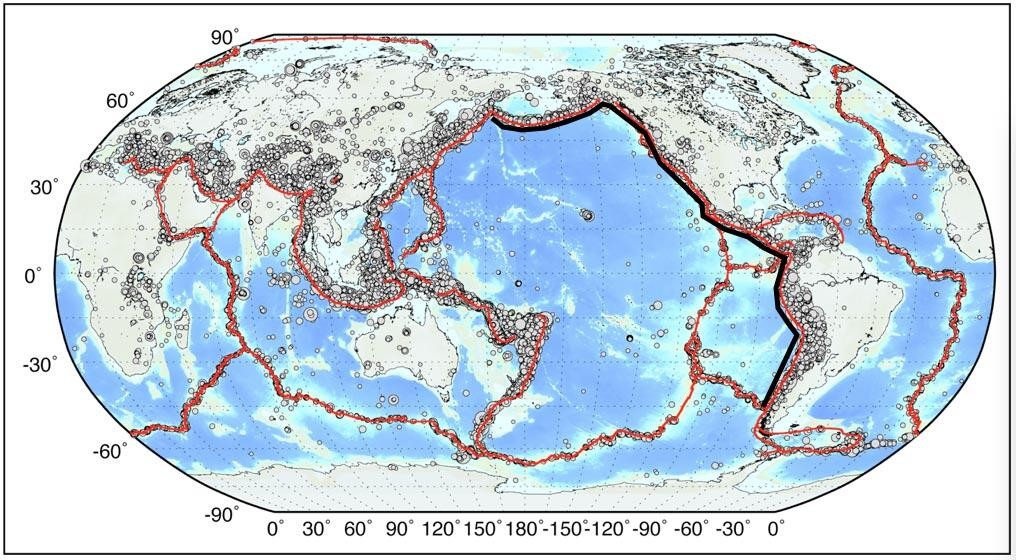
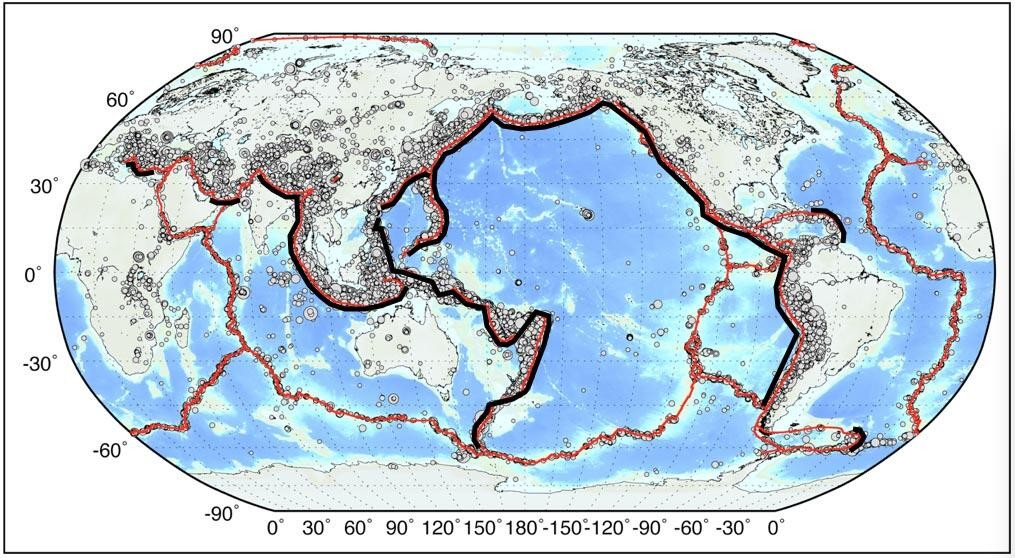
2. Unlocking Energy Release Insights
Graphs can also reveal how much energy an earthquake releases, which is crucial for understanding its potential for damage. The energy released is directly related to the magnitude, with each whole number increase in magnitude representing approximately 32 times more energy released. By examining these graphs, geologists can estimate the energy radiated by an earthquake and better assess the risk it poses to infrastructure and communities.
2.1 Calculating Energy Release
The energy released by an earthquake is a measure of the potential for damage to man-made structures. To compute an accurate value, all frequencies of shaking for the entire event must be included.
The relationship between magnitude and energy can be expressed as:
log E = 5.24 + 1.44Mw
Where E is the energy released (in joules) and Mw is the moment magnitude.
2.2 Visualizing Energy Release
Graphs illustrating energy release often show the relationship between magnitude and energy in a logarithmic scale. This allows geologists to quickly compare the energy released by earthquakes of different magnitudes.
For example, an earthquake with a magnitude of 6.0 releases approximately 32 times more energy than an earthquake with a magnitude of 5.0. This exponential relationship underscores the importance of accurate magnitude determination for assessing earthquake hazards.
3. Deciphering Shaking Intensity
While magnitude provides a single value for earthquake size, intensity measures the shaking experienced at different locations. Intensity values vary depending on distance from the fault rupture, local geology, and other factors. By analyzing intensity maps, geologists can understand the spatial distribution of shaking and identify areas at higher risk of damage.
3.1 Modified Mercalli Intensity Scale
The Modified Mercalli Intensity Scale is used in the United States to assess the intensity of shaking. It uses Roman numerals to represent different levels of shaking, ranging from I (not felt) to XII (catastrophic damage).
Intensity is traditionally a subjective measure derived from human observations and reports of felt shaking and damage. Today, this data is often collected through web-based forms like the “Did You Feel It?” system developed by the USGS. Instrumental data at each station location can also be used to calculate an estimated intensity.
3.2 Factors Affecting Intensity
Several factors can influence the intensity of shaking at a particular location:
- Distance from Fault Rupture: Intensity decreases with distance from the fault.
- Local Geology: Soft soils amplify shaking compared to bedrock.
- Earthquake Depth: Shallow earthquakes produce more intense shaking than deeper earthquakes.
- Rupture Directivity: The direction of the earthquake’s rupture can focus energy in certain areas.
4. Examples of Earthquake Analysis
4.1 Loma Prieta Earthquake (1989)
The Loma Prieta earthquake in 1989 demonstrated the influence of local geology on shaking intensity. Seismometers at similar distances from the epicenter recorded different shaking amplitudes depending on the underlying soil.
4.2 Northridge vs. Nisqually Earthquakes
The Northridge (CA) earthquake in 1994 and the Nisqually (WA) earthquake in 2001 had similar magnitudes, but the Northridge earthquake caused more intense shaking due to its shallower depth.
4.3 Importance of Depth
The depth of an earthquake plays a critical role in the intensity of shaking experienced at the surface. Shallower earthquakes tend to produce more intense shaking because the energy has less distance to travel before reaching the surface. This was evident in the comparison between the Northridge and Nisqually earthquakes.
5. Magnitude N Earthquake: Hypothetical Scenarios
5.1 Energy Release from All Earthquakes
If we sum the energy release from all earthquakes over the past ~110 years, the equivalent magnitude is ~Mw 9.95. This highlights the cumulative effect of numerous small and moderate earthquakes over time.
5.2 San Andreas Fault Rupture
If the San Andreas Fault were to rupture end-to-end (~1400km), with ~10m of average slip, it would produce an earthquake of Mw 8.47. This illustrates the potential for a major earthquake along one of the world’s most famous fault lines.
5.3 South American Subduction Zone Rupture
If the South American subduction zone were to rupture end-to-end (~6400km), with ~40m of average slip, it would produce an earthquake of Mw 9.86. This emphasizes the immense potential for large earthquakes in subduction zones.
5.4 Hypothetical Mw 10 Earthquake
To produce an Mw 10 earthquake, you would need ~14,000km fault length, with a seismogenic thickness averaging 40km (width of 100km), to slip an average of 30m.
5.5 Unlikely Mw 10.5 Earthquake
To produce an Mw 10.5 earthquake, you would need ~80,000km of fault length with an average seismogenic width of 100km. All of the subduction zones in the World, plus some adjoining structures amount to ~40,000km, and the circumference of the Earth is ~40,000km, so an Mw 10.5 is highly unlikely.
6. Advancements in Earthquake Monitoring
Advancement | Description | Impact |
---|---|---|
Real-time seismic networks | Networks of seismometers that continuously record and transmit data. | Enables rapid detection and characterization of earthquakes, improving early warning systems. |
GPS and InSAR technologies | Measure ground deformation associated with earthquakes. | Provides insights into fault behavior and stress accumulation, aiding in long-term hazard assessment. |
Advanced data processing techniques | Algorithms for analyzing seismic waveforms and extracting information about earthquake source parameters. | Improves the accuracy and precision of earthquake magnitude and location estimates. |
Machine learning and AI | Used for earthquake prediction, hazard assessment, and rapid damage assessment. | Enhances our ability to forecast earthquake occurrences, assess potential damage, and respond effectively to seismic events. |
Mobile apps and citizen science | Allows the public to report felt shaking and damage. | Provides valuable data on earthquake intensity and helps validate instrumental measurements. |
7. Earthquake Early Warning Systems
Earthquake early warning systems use real-time seismic data to detect earthquakes and send alerts to people in harm’s way before the arrival of strong shaking. These systems can provide seconds to minutes of warning, allowing people to take protective actions such as dropping, covering, and holding on.
7.1 How Early Warning Systems Work
- Detection: Seismic sensors detect the primary waves (P-waves) of an earthquake, which travel faster than the more destructive secondary waves (S-waves) and surface waves.
- Analysis: Algorithms analyze the P-wave data to estimate the earthquake’s location, magnitude, and potential shaking intensity.
- Alerting: Alerts are sent to users via mobile apps, public address systems, and other channels, providing a warning before the arrival of strong shaking.
7.2 Benefits of Early Warning Systems
- Personal Safety: Allows people to take protective actions during an earthquake.
- Infrastructure Protection: Enables automated shutdown of critical infrastructure such as gas lines and power grids.
- Reduced Damage: Provides time to secure objects and prevent damage to property.
8. Educational Resources at LEARNS.EDU.VN
At LEARNS.EDU.VN, we offer a wealth of resources to help you deepen your understanding of earthquake science and seismology. Our comprehensive materials cover topics ranging from the basics of earthquake magnitude and intensity to advanced techniques for analyzing seismic data. Whether you’re a student, educator, or simply someone with a passion for learning, you’ll find valuable information and engaging content to expand your knowledge.
8.1 Courses and Tutorials
Our courses and tutorials provide structured learning paths for exploring various aspects of earthquake science. You can learn about the different types of magnitude scales, the factors that influence shaking intensity, and the latest advancements in earthquake monitoring technology. Our interactive exercises and quizzes help you test your knowledge and reinforce your understanding.
8.2 Articles and Blog Posts
Our articles and blog posts cover a wide range of topics related to earthquakes and seismology. You can read about recent earthquake events, the science behind earthquake prediction, and the impact of earthquakes on society. Our content is written by experts in the field and is designed to be accessible to readers of all levels.
8.3 Expert Insights
LEARNS.EDU.VN provides access to insights from leading experts in seismology and earthquake science. You can learn from their research, perspectives, and experiences, gaining a deeper understanding of the challenges and opportunities in this exciting field.
9. Why Study Earthquake Science?
Studying earthquake science offers a range of benefits, both personally and professionally. It provides a deeper understanding of the natural world, enhances critical thinking skills, and opens up opportunities for careers in seismology, geology, and disaster management.
9.1 Personal Enrichment
Learning about earthquakes can help you better understand the risks in your area and take steps to protect yourself and your family. It can also foster a greater appreciation for the power and complexity of nature.
9.2 Career Opportunities
A background in earthquake science can lead to a variety of rewarding careers:
- Seismologist: Studies earthquakes and seismic waves to understand the Earth’s interior and assess earthquake hazards.
- Geologist: Investigates the Earth’s structure, composition, and processes, including earthquakes and faulting.
- Geophysicist: Uses physics to study the Earth, including seismic activity and ground deformation.
- Disaster Management Specialist: Develops and implements plans to mitigate the impacts of earthquakes and other natural disasters.
9.3 Contributing to Society
By studying earthquake science, you can contribute to society’s efforts to reduce earthquake risks and build more resilient communities. Your knowledge can help inform building codes, emergency response plans, and public awareness campaigns.
10. FAQ: Understanding Earthquake Graphs
Q1: What does the x-axis typically represent on an earthquake graph?
A1: The x-axis usually represents time, showing the progression of seismic waves as they are recorded by seismometers.
Q2: What does the y-axis typically represent on an earthquake graph?
A2: The y-axis typically represents the amplitude of the seismic waves, indicating the strength of the ground motion.
Q3: How can geologists determine the arrival times of different seismic waves from a graph?
A3: Geologists look for distinct changes in the graph’s pattern to identify the arrival times of P-waves, S-waves, and surface waves.
Q4: What information does the time difference between P-wave and S-wave arrivals provide?
A4: This time difference helps geologists determine the distance from the seismometer to the earthquake’s epicenter.
Q5: How is the magnitude of an earthquake estimated from a seismogram?
A5: The magnitude is estimated based on the amplitude of the seismic waves and the distance to the epicenter, using established magnitude scales like the Richter Scale or Moment Magnitude Scale.
Q6: Can a single earthquake graph provide all the information needed to analyze an earthquake?
A6: No, typically multiple seismograms from different locations are needed to accurately determine the location, depth, and magnitude of an earthquake.
Q7: What are some common patterns or features to look for on an earthquake graph?
A7: Look for sharp increases in amplitude indicating wave arrivals, variations in wave frequency, and the duration of shaking.
Q8: How do different types of soil or rock affect the appearance of an earthquake graph?
A8: Softer soils tend to amplify the amplitude of seismic waves, leading to larger peaks on the graph compared to recordings from seismometers on bedrock.
Q9: What are some limitations of using earthquake graphs to analyze earthquakes?
A9: Limitations include the potential for instrument errors, background noise that can obscure signals, and the complexity of interpreting graphs from earthquakes in areas with complex geology.
Q10: How do seismologists use advanced technologies like machine learning to analyze earthquake graphs?
A10: Machine learning algorithms can automatically identify and classify seismic events, detect subtle patterns that humans might miss, and improve the accuracy of earthquake location and magnitude estimates.
Conclusion: Empowering Your Understanding
Graphs are essential tools for geologists, providing insights into earthquake magnitude, energy release, and shaking intensity. By understanding how to interpret these graphs, you can gain a deeper appreciation for the dynamics of our planet and the risks associated with earthquakes. Whether you are looking to start a new skill, deepen your understanding of earthquakes, or pursue a career in earth science, LEARNS.EDU.VN has the resources and guidance you need.
Ready to unlock your potential and explore the world of earthquake science? Visit LEARNS.EDU.VN today and discover a wealth of educational content, expert insights, and interactive learning tools. Contact us at 123 Education Way, Learnville, CA 90210, United States, or reach out via Whatsapp at +1 555-555-1212. Start your journey of discovery with learns.edu.vn!